CellR4 2014; 2 (5): e1226
Human Stem Cell Exposure to Developmental Stage Zebrafish Extracts: a Novel Strategy for Tuning Stemness and Senescence Patterning
Topic: Stem cells
Category: Original Articles
Abstract
BACKGROUND: Zebrafish exhibits extraordinary ability for tissue regeneration. Despite growing investigations dissecting the molecular underpinning of such regenerative potential, little is known about the possibility to use the chemical inventory of the zebrafish embryo to modulate human stem cell dynamics.
METHODS: Extracts from zebrafish embryo were collected at different developmental stages, referred to as ZF1, ZF2, ZF3 (early stages), and ZF4, ZF5 (late stages). Human adipose-derived stem cells (hASCs), isolated from microfractured fat tissue obtained with a novel non-enzymatic method (Lipogems), were cultured in absence or presence of each developmental stage extract. Cell viability was assessed by MTT assay. Nuclear morphology was investigated by cell-permeable dye 4’,6-DAPI. Caspase-3 activity was assessed by ELISA. Gene transcription was monitored by real-time PCR.
RESULTS: Late developmental stage extracts decreased cell viability and elicited caspase-3 mediated apoptosis. This effect did not involve Bax or Bcl-2 transcription. Conversely, early developmental stage ZF1 did not affect cell viability or apoptosis, albeit increasing Bax/Bcl-2 mRNA ratio. ZF1 enhanced transcription of the stemness/pluripotency genes Oct-4, Sox-2 and c-Myc. ZF1 also induced the transcription of TERT, encoding the catalytic subunit of telomerase, as well as the gene expression of Bmi-1, a chromatin remodeler acting as a major telomerase-independent repressor of senescence. These transcriptional responses were restricted to the action of early stage factors, since they were not elicited by late developmental stage ZF5.
CONCLUSIONS: Exposure to early developmental stage zebrafish embryo extracts may enhance stem cell expression of multipotency and activate both telomerase-dependent and -independent antagonists of cell senescence. These outcomes may prove rewarding during prolonged expansion in culture, as it occurs in most cell therapy protocols.
Margherita Maioli, Federica Facchin, and Eva Bianconi equally contributed to the study
INTRODUCTION
Zebrafish maintains a remarkably higher ability than mammals to repair complex tissues after injury, including the heart and the central nervous system. For this reason, zebrafish embryos and their stem cells have been increasingly studied to unravel the molecular mechanisms underlying such regenerative potential, or to dissect evolutionary conserved pathways that may account for the regenerative action afforded by stem cells across different species.
Transplantation of human cord blood-derived CD34+ (hCD34+) cells into pregastrulation zebrafish embryos revealed that these human cells cosegregated with presumptive zebrafish hemangioblasts, being involved in early development of the embryonic vasculature of the recipient 1. Conversely, postgastrulation transplant resulted in the recruitment of hCD34+ cells into developing vessels, where their biology was mainly shifted to a paracrine action 2. These human cells were also found to accelerate vascular repair in adult zebrafish, after transplantation in a model of vascular regeneration induced by caudal fin amputation 3. These observations indicate unexpected developmental skills in human stem/progenitor cells and show that the possibility to modulate their differentiating and/or paracrine repertoire within the zebrafish embryo is tightly dependent from the microenvironmental context in a developmental stage-dependent fashion.
There is also growing evidence that embryonic development and tumorigenesis are closely correlated, as it can be inferred from the fact that they share several molecular pathways and regulatory molecules 4 , 5 , 6 , 7 , 8 , 9 , 10, and from the high tumorigenic risk associated with the acquirement of a pluripotent embryonic-like state, as it occurs during the preparation of human induced pluripotent stem cells 11 , 12. To this end, it has been shown that cell proliferation curves of different human cancer cell lines could be slowed down following exposure to zebrafish embryo extracts harvested during the stages of cell differentiation, with no significant antiproliferative effect in the presence of extracts taken from a merely duplicative stage 13 , 14 , 15 , 16 , 17. These observations also provide intriguing cues on the possibility to consider the tumorigenic process as a developmental deviation susceptible to control by regulators of cell differentiation, tracing a glimpse for future strategies of cancer (stem) cell reprogramming in the presence of differentiation stage factors produced by normal stem cells.
Despite the growing body of knowledge on the biology of the zebrafish embryo and stem cells, including the chance to use their secretome to impact on cancer cell dynamics, comparatively little is known about the possibility to exploit the chemical milieu provided at different developmental stages by the zebrafish embryo to modulate the homeostasis of human stem cells.
In the current study, we explored this novel perspective by exposing to zebrafish extracts, yielded at different developmental stages, human adipose-derived stem cells (hASCs), isolated from a microfractured fat tissue obtained with a novel non-enzymatic method and device (Lipogems) 18. We found that only the treatment with early developmental stage extracts was able to modulate the stem cell expression of multipotency, and elicited the transcriptional activation of two major mechanisms capable of counteracting stem cell senescence, including the gene expression of TERT, the catalytic subunit of telomerase, and the gene transcription of Bmi-1, a member of the Polycomb and Trithorax families group of repressors, acting as an essential factor for the self-renewal of adult stem cells, and as a key telomerase independent repressor of cell aging 19 , 20 , 21 , 22. Late, but not early developmental stage extracts induced a significant decrease in stem cell proliferation and the activation of pro-apoptotic signatures.
METHODS
Fat tissue processing, hASC harvesting and culture
According to the policies approved by the Institutional Review Boards for Human Studies local ethical committees, all tissue samples were obtained after informed consent. Human subcutaneous adipose tissue samples were obtained from lipoaspiration procedures and processed by using the Lipogems device, as previously described 23.
A volume of 1.5 ml of Lipogems product has been seeded in a T75 flask precoated with human fibronectin (0.55 μg/cm2) (Sigma-Aldrich Co., St. Louis, MO, USA) and human collagen I-III (0.50 μg/cm2) (ABCell-Bio), cultured in α-MEM medium supplemented with 20% heat-inactivated FBS, antibiotics (200 units/ml penicillin, 100 μg/ml streptomycin), L-Glutamine (1%), and incubated at 37°C in a humidified atmosphere with 5% CO2. Medium has been changed every 4 days, but the Lipogems product was maintained in culture for two weeks, then it was eliminated. At confluence, released cells were detached by treatment with trypsin-EDTA (Sigma-Aldrich Co., St. Louis, MO, USA), and subcultured. Experiments were performed at passage 3-5. All cell cultures were maintained 24 hours in standard conditions before treatments.
Zebrafish embryo extracts
Zebrafish embryos were harvested and processed as previously described 24 at 5 different developmental stages: 50% epiboly [5 hours post fertilization (hpf)], tail bud (10 hpf), 5 somites (12 hpf), 20 somites (19 hpf) and pharyngula (24 hpf), referred to as ZF1, ZF2, ZF3, ZF4 and ZF5, respectively. The embryos were washed in distilled water for 60 sec at the density of 800 eggs/ml. Extracts were prepared in a glycero-alcoholic solution (60% glycerol, 5% ethanol, 0.12% potassium sorbate and 0.08% sodium benzoate) and stored at 4°C until use. A 0.5% dilution of glycero-alcoholic solution in α-MEM with complements was used as a control in all experiments.
BCA protein assay
Protein content of each zebrafish embryo extract was determined with BCA protein assay kit, following the manufacturer’s instructions (Pierce Biotechnology, Rockford, IL, USA). A serial dilution of bovine serum albumin was used as standard, and NanoDrop (Nanodrop ND 1000 v.3.8.1, Wilmington, DE, USA) was used to determine the protein content of the extracts.
Cell viability
hASCs at the density of 5,000 cells/cm2 were cultured in a 24-well plate and incubated for 24 hours prior to starting the treatments. Cells were treated with ZF1, ZF2, ZF3, ZF4, or ZF5, each at four different concentrations: 0.01, 0.1, 1, or 10 μg/ml. As controls, hASCs were treated with a 0.5% glycero-alcoholic solution. The number of viable cells was determined after 24 and 72 hours of treatment, using the 3-(4, 5-dimethylthiazol-2-yl)2, 5-diphenyl-tetrazolium bromide (MTT, Sigma-Aldrich Co., St. Louis, MO, USA) assay. Briefly, at defined time points, cells were incubated for 3 hours with MTT in standard conditions (previously described) and subsequently lysed with a lysis buffer (90% iso-propanol, 10% TritonX-100 and 0.008% HCl). Absorbance of formazan salt was measured at 595 nm using a microplate reader (Opsys MR Microplate Reader; Dynex Technologies) and data were analyzed in GraphPad by one-way ANOVA followed by Dunnett’s post-hoc test. Each treatment was performed in triplicate, and the whole experiment was repeated in cells derived from at least three independent subjects.
Caspase-3 activity
To determine caspase-3 activity in hASCs treated in the absence or presence of ZF extracts, 10,000 cells/well were seeded in a 96-well black clear-bottom plate and cultured for 24 hours in standard conditions. Cells were treated with ZF4, or ZF5 at 0.1, 1, or 10 μg/ml for 72 hours. hASCs treated with a 0.5% glycero-alcoholic solution were used as control cells. Caspase-3 activity was determined using the Caspase-3 Colorimetric In-Cell ELISA Kit (Pierce Biotechnology, Rockford, IL, USA), following the manufacturer’s instructions, including staining with Janus Green as whole cell stain. Each treatment was performed in duplicate, and the whole experiment was repeated in cells derived from three independent subjects.
Assessment of nuclear morphology
To further assess cell apoptosis, hASCs were seeded at a density of 7,000 cells/cm2 onto coverslips placed in 24-well plates and maintained in standard conditions for 24 hours before treatments. Cells were treated with ZF4, or ZF5 at 10 μg/ml or with 0.5% glycero-alcoholic solution in α-MEM with complements as controls. After 72 hours, cells were fixed for 20 min using 4% formaldehyde, stained with UltraCruz Mounting medium for fluorescence with DAPI (cell-permeable dye 4’,6-DAPI) (Santa Cruz Biotechnology, Inc., Santa Cruz, CA, USA), and examined using a fluorescence microscope (Leitz Orthoplan; Leica DFC300 FX Digital Color Camera) to visualize the chromatin condensation and/or fragmentation typical for apoptotic cells. Each treatment was performed in duplicate, and the whole experiment was repeated in cells derived from three independent subjects. At least 200 cells, from different areas of the coverslips, were subjected to visual score for each sample.
RNA extraction and RT-PCR
hASCs were cultured in a 6-well plate at the density of 7,000 cells/cm2 and incubated for 24 hours before treatment. Cells were treated for 72 hours with the indicated ZF at 10 μg/ml or with 0.5% glycero-alcoholic solution in α-MEM with complements as a control. Each treatment was performed in duplicate, and the whole experiment was repeated in cells derived from three independent subjects.
Total RNA was extracted using the RNeasy Mini Kit (QIAGEN, Valencia, CA, USA) following the manufacturer’s instructions. RNA was subjected to reverse transcription (RT) using the following conditions: 2 µg total RNA, Moloney murine leukaemia virus reverse-transcriptase (Promega, Madison, WI; used with companion buffer) 400 U, oligo dT-15 2.5 µM, Random Hexamers 2 µM, dNTPs 500 µM each. RT reaction was performed in a final volume of 50 µl for 60 min at 37°C. In order to verify that the RT reaction was successful, amplification of the human Glyceraldehyde 3-phosphate dehydrogenase (GAPDH) gene was performed, using specific primers (Table 1). GAPDH amplification was performed in a final volume of 25 µl, using the following conditions; 1 µl cDNA, 0.2 µM each primer, 12.5 µl BioMix Red (Bioline, Taunton, MA); initial denaturation for 2 min at 94°C; 25 cycles of 30 s at 94°C, 30 s at 61°C (annealing temperature of GAPDH primers), 30 s at 72°C followed by a final extension for 7 min at 72°C. Amplicon detection was performed by gel electrophoresis in 1.5% agarose gel in TAE 1X (Merck) stained with 0.5 μg/ml Etidium Bromide (SIGMA) and visualized with UV-light.
Real-time PCR
Quantitative real-time PCR was performed using an iCycler Thermal Cycler (Bio-Rad). Two μl cDNA were amplified in 50-μl reactions using Platinum Supermix UDG (Invitrogen), 200 nM each primer, 10 nM fluorescein (Bio-Rad), and Sybr Green. After an initial denaturation step at 94°C for 10 min, temperature cycling was initiated. Each cycle consisted of 94°C for 15 s, 55-59°C for 30 s and 60°C for 30 s, the fluorescence being read at the end of this step. The primers used were specific and spanning exons. Their list is reported in Table 1.
To evaluate the quality of product of real-time PCR assays, melting curve analysis was performed after each assay. Relative expression was determined using the “delta-CT method”25 with hypoxanthine phosphoribosyltransferase 1 (HPRT1) as a reference gene.
Data analysis
Data are presented as mean ± standard deviation. As indicated in the legend of each figure, the statistical analysis was determined by one-way ANOVA followed by Dunnett’s post-hoc test, or by two-tailed unpaired Student’s t test. A p value less than 0.05 was assumed as the limit of significance.
RESULTS
Late- but not early-developmental stage extracts from zebrafish embryo reduce hASC viability
We first investigated whether hASC exposure to embryo extracts collected at different developmental stages may differentially affect cell viability. Cell culture in the presence of ZF4 or ZF5 only produced a slight decrease in the yield of viable cells after 24 hours (Figure 1, A), but resulted in a significant, dose-dependent reduction in the number of viable elements after 72 hours of exposure (Figure 1, B). No effect on cell viability was detected after treatment for 24 or 72 hours with early developmental stage embryo extracts, including ZF1, ZF2 or ZF3 (Figure 1, A and B).
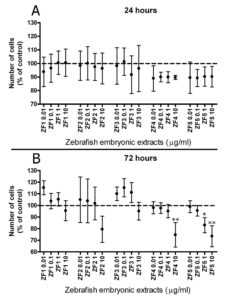
Figure 1. Comparative analysis of stem cell viability in the presence of early or late developmental stage zebrafish extracts. Cell viability was evaluated by MTT proliferation assay in control and hASCs exposed to ZF1, ZF2, ZF3, ZF4, and ZF5. Each stage related extract was given at the final concentrations of 0.01, 0.1, 1, and 10 μg/ml, for 24 hours (Panel A) or 72 hours (Panel B). Data are expressed as mean ± standard deviation of three independent experiments performed in duplicate on hASCs derived from three independent subjects. Cell viability is significantly reduced in ZF4 (10 μg/ml), and ZF5 (1 and 10 μg/ml) treated cells compared with control cells after 72 hours. Statistical analysis was determined by one-way ANOVA followed by Dunnett’s post-hoc test (*p < 0.05, **p < 0.01).
We next investigated whether the reduced cell viability observed after treatment with late developmental stage extracts may be due to an increase in cell apoptosis. As shown in Figure 2, caspase-3 activity was significantly increased in cells treated with ZF4 or ZF5 at 10 μg/ml for 72 hours, as compared with the control cells. Caspase-3 activity was not affected by ZF1, ZF2 or ZF3 (not shown).
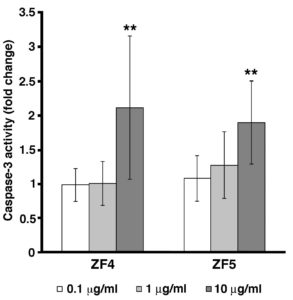
Figure 2. Caspase-3 activity is induced by late stage developmental extracts. Caspase-3 activity was evaluated in control and exposed cells by ELISA assay. hASCs were treated with ZF4, or ZF5 at the concentrations of 0.1, 1, and 10 μg/ml for 72 hours. Data are presented as ratio of treated cells to control cells (fold change). Caspase-3 activity is significantly increased in both ZF4 (10 μg/ml) and ZF5 (10 μg/ml) treated cells, as compared with control cells (**p < 0.01). Data are expressed as mean ± standard deviation of three independent experiments performed in duplicate on hASCs derived from three independent subjects. Statistical analysis was determined by t-test method.
The induction of an apoptotic trait following exposure to late developmental stage extracts was further inferred by the analysis of nuclear morphology showing evident chromatin condensation and increased percentage of apoptotic cells in the presence of 10 μg/ml ZF4 or ZF5, as compared to unexposed hASCs (Figure 3).
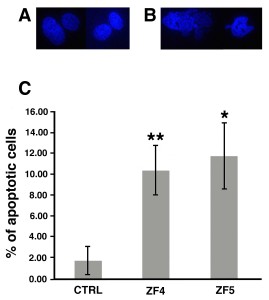
Figure 3. Percentage assessment of apoptotic cells. Apoptosis was evaluated by DAPI staining in control untreated cells and in hASCs that had been treated for 72 hours with ZF4, or ZF5 (10 μg/ml). A and B, Representative images of nuclear morphology in control and treated hASCs, respectively. C, Percentage changes in the amount of apoptotic cells. Data are expressed as mean ± standard deviation of three independent experiments performed in duplicate on hASCs derived from three independent subjects. Nuclear morphology highlights a significant increase of apoptosis in all treated cells, as compared with control (CTRL) hASCs (*p < 0.05, **p < 0.01). Statistical analysis was determined by t-test method.
Gene expression analysis revealed that hASC treatment with ZF1 for 72 hours enhanced Bax transcription (Figure 4, A) along with a downregulation in the expression of Bcl-2 mRNA (Figure 4, B), encoding a major prosurvival player 26. On the other hand, exposure to the late stage ZF5 did not affect significantly both Bax and Bcl-2 transcription (Figure 4, A and B).
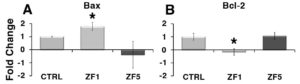
Figure 4. Analysis of the effect of early and late developmental stage factors on the gene transcription of apoptotic modulators. The gene expression of Bax or Bcl-2 was evaluated by real-time PCR in controls and hASCs that had been cultured for 72 hours in the presence of 10 μg/ml ZF 1, or ZF5. The amount of Bax (A) or Bcl-2 (B) mRNA from control (CTRL) and treated hASCs (ZF1 or ZF5) was normalized to HPRT1 and was plotted as fold change relative to the mRNA expression of control untreated cells, defined as 1. Data are expressed as mean ± standard deviation of three independent experiments performed in duplicate on hASCs derived from three independent subjects. * Significantly different from the control (p < 0.05). Statistical analysis was determined by t-test method.
Early-, but not late-developmental stage embryo extracts enhance the expression of stemness related genes and activate the transcription of antisenescence orchestrators
Real-time PCR shown that hASC treatment with the early developmental stage ZF1 for 72 hours was able to remarkably increase the transcription of Oct-4 and Sox-2 (Figure 5, A and B). The transcription of both genes was not affected by the late developmental stage ZF5 (Figure 5, A and B). Similar to Oct-4 and Sox-2 expression, the transcription of c-Myc was upregulated by ZF1, while being unaffected by ZF5 (Figure 5, C).
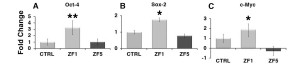
Figure 5. Early developmental stage extracts enhance the transcription of stemness/pluripotency-related genes. The gene expression of Oct-4 (A), Sox-2 (B) or c-Myc (C) was evaluated by real-time PCR in untreated cells and hASCs exposed for 72 hours to ZF1, or ZF5 (10 μg/ml). The amount of the investigated mRNAs from control (CTRL) and treated hASCs (ZF1 or ZF5) was normalized to HPRT1 and was plotted as fold change relative to the mRNA expression of control untreated cells, defined as 1. Data are expressed as mean ± standard deviation of three independent experiments performed in duplicate on hASCs derived from three independent subjects. Significantly different from the control: *p < 0.05, **p < 0.01. Statistical analysis was determined by t-test method.
Exposure of hASCs in the presence of ZF1 led to a consistent overexpression of the TERT gene, encoding the catalytic subunit of telomerase (Figure 6, A). The gene expression of Bmi-1, a transcriptional regulator involved in chromatin remodeling and acting as a telomerase-independent repressor of senescence, was also increased by ZF1 (Figure 6, B). Stem cell treatment with ZF5 did not modify TERT transcription, but significantly reduced Bmi-1 gene expression (Figure 6, A and B).
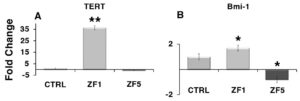
Figure 6. Early developmental stage factors promote the transcriptional activation of two major antagonists of cell senescence. The gene expression of TERT (A) or Bmi-1 (B) was evaluated by real-time PCR in untreated cells and hASCs exposed for 72 hours to ZF 1, or ZF5 (10 μg/ml). The amount of the investigated mRNAs from control (CTRL) and treated hASCs (ZF1 or ZF5) was normalized to HPRT1 and was plotted as fold change relative to the mRNA expression of control untreated cells, defined as 1. Data are expressed as mean ± standard deviation of three independent experiments performed in duplicate on hASCs derived from three independent subjects. Significantly different from the control: *p < 0.05, **p < 0.01. Statistical analysis was determined by t-test method.
DISCUSSION
In the last few years, a growing body of studies has been designed to exploit the rescuing potential of human mesenchymal stem cells (hMSCs) for the treatment of injured tissues. Although hMSCs have been isolated from many different tissues, their number is exiguous in all tissue sources. Meta-analysis of currently available cell therapy protocols shows that hMSCs are transplanted at high doses, between 10 and 400 million hMSCs per treatment (www.clinicaltrials.gov). The needs for expansion is particularly envisionable in protocols based on intravascular delivery of stem cells, that cannot be transplanted in form of tissue extracts (i.e. processed fat tissue). To fulfill these requirements, hMSCs undergo multiple passages and prolonged time in culture, usually 8-12 weeks. This approach has been shown to be both a risk of, and a well-established model for cell aging in vitro 27 , 28. Moreover, prolonged expansion impairs stem cell expression of pluripotency/multipotency, leading to a consistent decline in the multilineage repertoire and in the yield of differentiated cells.
hASCs are now being used as an easy-to-harvest tool for cell therapy, and exhibit phenotypic and transcriptional profiles similar to hMSCs, as well as robust multilineage potential in vitro. Despite these attractive features, hASCs also undergo significant senescence and decline in multipotency expression after multiple passages in culture 29 , 30 , 31. These findings raise cautionary notes whenever long passaged hASCs are used in a clinical setting, and prompt the needs for novel approaches that may oppose senescence and optimize the expression of multipotency in such a promising tool for cell therapy.
Here, we have exposed hASCs in the presence of zebrafish extracts harvested at different developmental stages, showing that only the late developmental stage extracts (ZF4 and 5) significantly decreased cell proliferation and viability. The ZF4/ZF5 effect involved the activation of a proapoptotic program, as shown by the derangement in nuclear morphology and the chromatin condensation, and by the activation of caspase-3. The finding that ZF5 failed to affect the transcription of Bax and Bcl-2, two major players in the modulation of apoptosis, suggests that apoptosis mediated by late stage developmental factors of zebrafish embryo is Bax-independent. This phenomenon has long been observed, as shown in the case of Bax-independent, caspase-3-related apoptosis induced by HGF in rat liver epithelial cells 32, and recently confirmed in both malignant and normal cells 33 , 34.
Unlike ZF5, ZF1-3 did not induce hASC apoptosis, nor they decreased cell viability. This different behaviour may result from a fine equilibrium between ZF1-induced transcription of Oct-4, Sox-2, TERT, Bmi-1 and c-Myc, which have all been found to inhibit apoptotic pathways 35 , 36 , 37 , 38 , 39 40, and the increase in Bax/Bcl-2 mRNA ratio observed in ZF1-exposed hASCs. This hypothesis is consonant with the emerging view that crucial modulators of apoptotic pathways do not behave as on-off transcriptional specifiers, but they rather act as non-linear boosters of the expression of active genes, based upon the adjustments in the settings of critical thresholds resulting from the inventory of regulatory players in given cell populations 41. To this end, c-Myc has also been found to act as a positive regulator of apoptosis in human embryonic stem cells 42 , 43. Further studies are needed to trace a proteomic profiling of early and late developmental stage zebrafish extracts and possibly screen factors that could specifically induce caspase activation and the release of cytocrome-c in a Bax-dependent or -independent fashion.
During zebrafish embryogenesis the expression of the pluripotency genes Pou5f1/Oct-4 and Sox-2 is timely regulated by defined factors that are mainly restricted to the early developmental pattern (during the first hpf) 44 , 45 , 46. The current data show that hASCs are able to selectively respond to factors restricted to the very early, but not late, developmental stages of zebrafish embryo with a transcriptional increase in the same two stemness-related genes necessary for the expression of family members of transcription factors that contribute to the maintenance of human stem cell pluripotency and self-renewal. We have previously shown that hASCs overexpressing Oct-4 and Sox-2 following exposure to an asymmetrically conveyed radioelectric field exhibited enhanced commitment towards cardiac, vascular, skeletal muscle, and neuronal lineages, with higher differentiating yields from Lipogems-derived hASCs than cells obtained from enzymatic digestion of the same lipoaspirate 47. Whether the currently observed increase of Oct-4 and Sox-2 in response to targeted zebrafish embryo extracts may also lead to enhanced multilineage commitment in vitro and rescuing potential in vivo remains to be established, and it is the subject of ongoing investigations.
We show that the overexpression of stemness genes elicited by ZF1 was paralleled by an increase in the transcription of both Bmi-1 and TERT. The relevance of this observation is highlighted by the fact that both genes exert a major role in counteracting aging processes in vivo and cell senescence in vitro. Bmi-1 is emerging as a major aging repressor and is transcriptionally down-regulated when cells undergo replicative senescence 48 , 49 , 50. TERT opposes cell senescence by counteracting telomere shortening. Studies on brain development in mice have correlated a decrease in TERT expression and activity with decreased neuroblast proliferation, and differentiation 51. Moreover, it has been demonstrated that MSCs or bone marrow stromal stem cells lacking telomerase activity undergo premature cellular senescence, with a progressive decline in the expression of early mesenchymal stem cell markers 52. The maintenance of stemness gene expression is also important in the prevention of cell senescence. The singular loss of the Bright/Arid3A transcription factor, the founding member of the ARID family of transcriptions factors 53 , 54, which binds directly to the promoter/enhancer regions of Oct-4 and Sox-2 contributing to their repression in both mouse embryonic fibroblasts (MEFs) and mouse embryonic stem cells (ESCs), was found to bypass the cell senescene barrier, leading to MEF reprogramming 55.
CONCLUSIONS
We show for the first time that human stem cell exposure to early developmental stage zebrafish embryo extracts may represent a useful tool to enhance stem cell expression of multipotency and activate both telomerase-dependent and -independent antagonists of cell senescence. This strategy did not require cumbersome gene manipulation through viral vector mediated gene transfer, or expensive synthetic chemistry. Further studies are in progress to investigate whether developmental stage extracts from zebrafish embryo may be used to revert cell senescence in hMSCs subjected to expansion for multiple passages in vitro, resuming their ability to differentiate along multiple lineages.
Acknowledgments
This research was supported by Wartsila Italia Spa, Trieste, Italy; Ettore Sansavini Health Science Foundation, Italy; Ministero della Salute, Italy, Ricerca Finalizzata-Progetti Cellule Staminali 2008, Italy.
The Foundation BLANCEFLOR Boncompagni Ludovisi, née Bildt; The C.M. Lerici Foundation.
Conflict of Interests:The Authors declare that they have no conflict of interests.
References
- Pozzoli O, Vella P, Iaffaldano G, Parente V, Devanna P, Lacovich M, Lamia CL, Fascio U, Longoni D, Cotelli F, Capogrossi MC, Pesce M. Endothelial fate and angiogenic properties of human CD34+ progenitor cells in zebrafish. Arterioscler Thromb Vasc Biol 2011; 31: 1589-1597. (back)
- Pozzoli O, Vella P, Iaffaldano G, Parente V, Devanna P, Lacovich M, Lamia CL, Fascio U, Longoni D, Cotelli F, Capogrossi MC, Pesce M. Endothelial fate and angiogenic properties of human CD34+ progenitor cells in zebrafish. Arterioscler Thromb Vasc Biol 2011; 31: 1589-1597. (back)
- Pozzoli O, Vella P, Iaffaldano G, Parente V, Devanna P, Lacovich M, Lamia CL, Fascio U, Longoni D, Cotelli F, Capogrossi MC, Pesce M. Endothelial fate and angiogenic properties of human CD34+ progenitor cells in zebrafish. Arterioscler Thromb Vasc Biol 2011; 31: 1589-1597. (back)
- Biava PM, Bonsignorio D. Cancer and cell differentiation: a model to explain malignancy. J Tumor Marker Oncol 2002; 17: 47-54. (back)
- Biava PM, Nicolini A, Ferrari P, Carpi A, Sell S. A systemic approach to cancer treatment: tumor cell reprogramming focused on endocrine-related cancers. Curr Med Chem 2014; 21: 1072-1081. (back)
- Minz B, Illmensee K. Normal genetically mosaic mice produced from malignant teratocarcinoma cells. Proc Natl Acad Sci USA1975; 72: 3585-3589. (back)
- Papaioannou VE, McBumey MV, Gardner RL, Evans RL. Fate of teratocarcinoma cells injected into early mouse embryos. Nature 1975; 258: 70-73. (back)
- Pierce GB. The cancer cell and its control by the embryo. Am J Pathol 1983; 113: 116-124. (back)
- Hendrix MJ, Seftor EA, Seftor REB, Kaisermeier-Kulesa J, Kulesa PM, Postovit LM. Reprogramming metastatic tumor cells with embryonic microenvironment. Nat Rev Cancer 2007; 7: 246-255. (back)
- Postovit LM, Maragaryan NV, Seftor EA, Kirschmann DA, Lipavski A, Wheaton WW, Abbott DE, Seftor RE, Hendrix MJ. Human embryonic stem cell microenvironment suppress the tumorigenic phenotype of aggressive cancer cells. Proc Natl Acad Sci USA 2008; 18: 105-111. (back)
- Ohnishi K, Semi K, Yamada Y. Epigenetic regulation leading to induced pluripotency drives cancer development in vivo. Biochem Biophys Res Commun 2014 Jul 11. pii: S0006-291X(14)01234-0. doi: 10.1016/j.bbrc.2014.07.020. [Epub ahead of print]. (back)
- Ohnishi K, Semi K, Yamamoto T, Shimizu M, Tanaka A, Mitsunaga K, Okita K, Osafune K, Arioka Y, Maeda T, Soejima H, Moriwaki H, Yamanaka S, Woltjen K, Yamada Y. Premature termination of reprogramming in vivo leads to cancer development through altered epigenetic regulation. Cell 2014; 156: 663-677. (back)
- Biava PM, Bonsignorio D, Hoxa M. Cell proliferation curves of different human tumor lines after in vitro treatment with Zebrafish embryonic extracts. J Tumor Marker Oncol 2001; 16: 195-202. (back)
- Biava PM, Bonsignorio D, Hoxa M, Facco R, Ielapi T, Frati L, Bizzarri, M. Post-traslational modification of the retinoblastoma protein (pRb) induced by in vitro administration of Zebrafish embryonic extracts on human kidney adenocarcinoma cell line. J Tumor Marker Oncol 2002; 17: 59-64. (back)
- Cucina A, Biava PM, D’Anselmi F, Coluccia P, Conti F, Di Clemente R, Miccheli A, Frati L, Gulino A, Bizzani M. Zebrafish embryo proteins induce apoptosis in human colon cancer cells (Caco2). Apoptosis 2006; 9: l617-1628. (back)
- Biava PM, Basevi M, Biggiero L, Borgonovo A, Borgonovo E, Burigana F. Cancer cell reprogramming: stem cell differentiation stage factors and an agent based model to optimize cancer treatment. Curr Pharm Biotechnol 2011; 12: 231-242. (back)
- Livraghi T, Meloni F, Frosi A, Lazzaroni S, Bizzani M, Frati L, Biava PM. Treatment with stem cell differentiation stage factors of intermediate-advanced hepatocellular carcinoma: an open randomized clinical trial. Oncol Res 2005; 15: 399-408. (back)
- Bianchi F, Maioli M, Leonardi E, Olivi E, Pasquinelli G, Valente S, Mendez AJ, Ricordi C, Raffaini M, Tremolada C, Ventura C. A new nonenzymatic method and device to obtain a fat tissue derivative highly enriched in pericyte-like elements by mild mechanical forces from human lipoaspirates. Cell Transplant 2013; 22: 2063-2077. (back)
- Molofsky AV, Pardal R, Iwashita T, Park IK, Clarke MF, Morrison SJ. Bmi-1 dependence distinguishes neural stem cell self-renewal from progenitor proliferation. Nature 2003; 425: 962-967. (back)
- Guney I, Sedivy JM. Cellular senescence, epigenetic switches and c-Myc. Cell Cycle 2006; 5: 2319-2323. (back)
- Lessard J, Sauvageau G. Bmi-1 determines the proliferative capacity of normal and leukaemic stem cells. Nature 2003; 423: 255-260. (back)
- Park IK, Qian D, Kiel M, Becker MW, Pihalja M, Weissman IL, Morrison SJ, Clarke MF. Bmi-1 is required for maintenance of adult self-renewing haematopoietic stem cells. Nature 2003; 423: 302-305. (back)
- Bianchi F, Maioli M, Leonardi E, Olivi E, Pasquinelli G, Valente S, Mendez AJ, Ricordi C, Raffaini M, Tremolada C, Ventura C. A new nonenzymatic method and device to obtain a fat tissue derivative highly enriched in pericyte-like elements by mild mechanical forces from human lipoaspirates. Cell Transplant 2013; 22: 2063-2077. (back)
- Livraghi T, Meloni F, Frosi A, Lazzaroni S, Bizzani M, Frati L, Biava PM. Treatment with stem cell differentiation stage factors of intermediate-advanced hepatocellular carcinoma: an open randomized clinical trial. Oncol Res 2005; 15: 399-408. (back)
- Pfaffl MW. A new mathematical model for relative quantification in real-time RTPCR. Nucleic Acids Res 2001; 29: e45. (back)
- Kelly PN, Strasser A. The role of Bcl-2 and its pro-survival relatives in tumourigenesis and cancer therapy. Cell Death Differ 2011; 18: 1414-1424. (back)
- Estrada JC, Torres Y, Benguría A, Dopazo A, Roche E, Carrera-Quintanar L, Pérez RA, Enríquez JA, Torres R, Ramírez JC, Samper E, Bernad A. Human mesenchymal stem cell-replicative senescence and oxidative stress are closely linked to aneuploidy. Cell Death Dis 2013; 4: e691. (back)
- Izadpanah R, Kaushal D, Kriedt C, Tsien F, Patel B, Dufour J, Bunnell BA. Long-term in vitro expansion alters the biology of adult mesenchymal stem cells. Cancer Res 2008; 68: 4229-4238. (back)
- Estrada JC, Torres Y, Benguría A, Dopazo A, Roche E, Carrera-Quintanar L, Pérez RA, Enríquez JA, Torres R, Ramírez JC, Samper E, Bernad A. Human mesenchymal stem cell-replicative senescence and oxidative stress are closely linked to aneuploidy. Cell Death Dis 2013; 4: e691. (back)
- Gruber HE, Somayaji S, Riley F, Hoelscher GL, Norton HJ, Ingram J, Hanley EN Jr. Human adipose-derived mesenchymal stem cells: serial passaging, doubling time and cell senescence. Biotech Histochem 2012; 87: 303-311. (back)
- Han SM, Han SH, Coh YR, Jang G, Chan Ra J, Kang SK, Lee HW, Youn HY. Enhanced proliferation and differentiation of Oct4- and Sox2-overexpressing human adipose tissue mesenchymal stem cells. Exp Mol Med 2014; 46: e101. (back)
- Conner EA, Teramoto T, Wirth PJ, Kiss A, Garfield S, Thorgeirsson SS. HGF-mediated apoptosis via p53/bax-independent pathway activating JNK1. Carcinogenesis 1999; 20: 583-590. (back)
- Mullauer FB, Kessler JH, Medema JP. Betulinic acid induces cytochrome c release and apoptosis in a Bax/Bak-independent, permeability transition pore dependent fashion. Apoptosis 2009; 14: 191-202. (back)
- Lei X, Chen Y, Du G, Yu W, Wang X, Qu H, Xia B, He H, Mao J, Zong W, Liao X, Mehrpour M, Hao X, Chen Q. Gossypol induces Bax/Bak-independent activation of apoptosis and cytochrome c release via a conformational change in Bcl-2. FASEB J 2006; 20: 2147-2149. (back)
- Wen K, Fu Z, Wu X, Feng J, Chen W, Qian J. Oct-4 is required for an antiapoptotic behavior of chemoresistant colorectal cancer cells enriched for cancer stem cells: effects associated with STAT3/Survivin. Cancer Lett 2013; 333: 56-65. (back)
- Lin Y, Yang Y, Li W, Chen Q, Li J, Pan X, Zhou L, Liu C, Chen C, He J, Cao H, Yao H, Zheng L, Xu X, Xia Z, Ren J, Xiao L, Li L, Shen B, Zhou H, Wang YJ. Reciprocal regulation of Akt and Oct4 promotes the self-renewal and survival of embryonal carcinoma cells. Mol Cell 2012; 48: 627-640. (back)
- Thiel G. How Sox2 maintains neural stem cell identity. Biochem J 2013; 450: e1-2. (back)
- Feng R, Zhou S, Liu Y, Song D, Luan Z, Dai X, Li Y, Tang N, Wen J, Li L. Sox2 protects neural stem cells from apoptosis via up-regulating survivin expression. Biochem J 2013; 450: 459-468. (back)
- Madonna R, Taylor DA, Geng YJ, De Caterina R, Shelat H, Perin EC, Willerson JT. Transplantation of mesenchymal cells rejuvenated by the overexpression of telomerase and myocardin promotes revascularization and tissue repair in a murine model of hindlimb ischemia. Circ Res 2013; 113: 902-914. (back)
- Lüscher B, Vervoorts J. Regulation of gene transcription by the oncoprotein MYC. Gene 2012; 494: 145-160. (back)
- Nie Z, Hu G, Wei G, Cui K, Yamane A, Resch W, Wang R, Green DR, Tessarollo L, Casellas R, Zhao K, Levens D. c-Myc is a universal amplifier of expressed genes in lymphocytes and embryonic stem cells. Cell 2012; 151: 68-79. (back)
- Nie Z, Hu G, Wei G, Cui K, Yamane A, Resch W, Wang R, Green DR, Tessarollo L, Casellas R, Zhao K, Levens D. c-Myc is a universal amplifier of expressed genes in lymphocytes and embryonic stem cells. Cell 2012; 151: 68-79. (back)
- Sumi T, Tsuneyoshi N, Nakatsuji N, Suemori H. Apoptosis and differentiation of human embryonic stem cells induced by sustained activation of c-Myc. Oncogene 2007; 26: 5564-5576. (back)
- Lunde K, Belting HG, Driever W. Zebrafish pou5f1/pou2, homolog of mammalian Oct4, functions in the endoderm specification cascade. Curr Biol 2004; 14: 48-55. (back)
- Onichtchouk D, Geier F, Polok B, Messerschmidt DM, Mössner R, Wendik B, Song S, Taylor V, Timmer J, Driever W. Zebrafish Pou5f1-dependent transcriptional networks in temporal control of early development. Mol Syst Biol 2010; 6: 354. (back)
- Kotkamp K, Mössner R, Allen A, Onichtchouk D, Driever W. A Pou5f1/Oct4 dependent Klf2a, Klf2b, and Klf17 regulatory sub-network contributes to EVL and ectoderm development during zebrafish embryogenesis. Dev Biol 2014; 385: 433-447. (back)
- Maioli M, Rinaldi S, Santaniello S, Castagna A, Pigliaru G, Delitala A, Bianchi F, Tremolada C, Fontani V, Ventura C. Radio electric asymmetric conveyed fields and human adipose-derived stem cells obtained with a non-enzymatic method and device: a novel approach to multipotency. Cell Transplant 2013 Aug 30. doi: 10.3727/096368913X672037. [Epub ahead of print]. (back)
- Molofsky AV, Pardal R, Iwashita T, Park IK, Clarke MF, Morrison SJ. Bmi-1 dependence distinguishes neural stem cell self-renewal from progenitor proliferation. Nature 2003; 425: 962-967. (back)
- Bianco P, Riminucci M, Gronthos S, Robey PG. Bone marrow stromal stem cells: nature, biology, and potential applications. Stem Cells 2001; 19: 180-192. (back)
- Rando TA. Stem cells, ageing and the quest for immortality. Nature 2006; 441: 1080-1086. (back)
- Itahana K, Zou Y, Itahana Y, Martinez JL, Beausejour C, Jacobs JJ, Van Lohuizen M, Band V, Campisi J, Dimri GP. Control of the replicative life span of human fibroblasts by p16 and the polycomb protein Bmi-1. Mol Cell Biol 2003; 23: 389-401. (back)
- Klapper W, Parwaresch R, Krupp G. Telomere biology in human aging and aging syndromes. Mech Ageing Dev 2001; 122: 695-712. (back)
- Herrscher RF, Kaplan MH, Lelsz DL, Das C, Scheuermann R, Tucker PW. The immunoglobulin heavy-chain matrix-associating regions are bound by Bright: a B cell-specific trans-activator that describes a new DNA-binding protein family. Genes Dev 1995; 9: 3067-3082. (back)
- Wilsker D, Patsialou A, Dallas PB, Moran E. ARID proteins: a diverse family of DNA binding proteins implicated in the control of cell growth, differentiation, and development. Cell Growth Differ 2002; 13: 95-106. (back)
- Popowski M, Templeton TD, Lee BK, Rhee C, Li H, Miner C, Dekker JD, Orlanski S, Bergman Y, Iyer VR, Webb CF, Tucker H. Bright/Arid3A Acts as a Barrier to Somatic Cell Reprogramming through Direct Regulation of Oct4, Sox2, and Nanog. Stem Cell Reports 2014; 2: 26-35. (back)
To cite this article
Human Stem Cell Exposure to Developmental Stage Zebrafish Extracts: a Novel Strategy for Tuning Stemness and Senescence Patterning
CellR4 2014; 2 (5): e1226
Publication History
Published online: 30 Sep 2014

This work is licensed under a Creative Commons Attribution-NonCommercial-ShareAlike 4.0 International License.