CellR4 2014; 2 (2): e845
The Cardiac Regenerative Potential of Myoblasts Remains Limited Despite Improving their Survival Via Antioxidant Treatment
Category: Original Articles
Abstract
INTRODUCTION: Since myoblasts have been limited by poor cell survival after cellular myoplasty, the major goal of the current study was to determine whether improving myoblast survival with an antioxidant could improve cardiac function after the transplantation of the myoblasts into an acute myocardial infarction.
BACKGROUND: We previously demonstrated that early myogenic progenitors such as muscle-derived stem cells (MDSCs) exhibited superior cell survival and improved cardiac repair after transplantation into infarcted hearts compared to myoblasts, which we partially attributed to MDSC’s higher antioxidant levels.
AIM: To determine if antioxidant treatment could increase myoblast survival, subsequently improving cardiac function after myoblast transplantation into infarcted hearts.
MATERIALS AND METHODS: Myoblasts were pre-treated with the antioxidant N-acetylcysteine (NAC) or the glutathione depleter, diethyl maleate (DEM), and injected into infarcted murine hearts. Regenerative potential was monitored by cell survival and cardiac function.
RESULTS: At early time points, hearts injected with NAC-treated myoblasts exhibited increased donor cell survival, greater cell proliferation, and decreased cellular apoptosis, compared to untreated myoblasts. NAC-treated myoblasts significantly improved cardiac contractility, reduced fibrosis, and increased vascular density compared to DEM-treated myoblasts, but compared to untreated myoblasts, no difference was noted.
DISCUSSION: While early survival of myoblasts transplanted into infarcted hearts was augmented by NAC pre-treatment, cardiac function remained unchanged compared to non-treated myoblasts.
CONCLUSIONS: Despite improving cell survival with NAC treated myoblast transplantation in a MI heart, cardiac function remained similar to untreated myoblasts. These results suggest that the reduced cardiac regenerative potential of myoblasts, when compared to MDSCs, is not only attributable to cell survival but is probably also related to the secretion of paracrine factors by the MDSCs.
Introduction
Cell therapy is a promising approach to treat heart disease, which in the United States alone is the cause of 1 in every 2.8 deaths 1. Benefits of stem/progenitor cell therapy result largely from increased angiogenesis 2, decreased apoptosis of native cardiomyocytes, decreased fibrosis 3 and increased contractile properties of the left ventricle (LV); however, low engraftment and survival of donor cells 4 remains a universal obstacle to cell therapy 5 , 6. The harsh microenvironment that exists in the heart after MI leads to the accumulation of high levels of inflammatory and oxidative stressors, which can cause irreversible damage to the transplanted donor cells 7 , 8; therefore, the development of methods to improve cell survival and overcome environmental stressors would be beneficial for cellular myoplasty.
Background
Muscle-derived stem cells (MDSCs) represent a subpopulation of muscle cells that are isolated from skeletal muscle based upon their low adhesion to collagen coated flasks 9 , 10 , 11. We previously demonstrated that MDSCs promoted cardiac and skeletal muscle repair in a more effective manner than myoblasts, likely due to the superior cell survival of the MDSCs 12 , 13. Greater survival of MDSCs under oxidative stress, compared to myoblasts, was attributed to their higher intracellular levels of the antioxidant glutathione (GSH) and enzyme superoxide dismutase (SOD) 14. In support of this, pre-treating MDSCs with DEM to deplete antioxidant levels prior to cardiac implantation resulted in decreased LV contractile function, decreased angiogenesis, and increased fibrosis, which was similar to what was observed in PBS injected hearts 15 , 16. Alternatively, N-acetylcysteine (NAC), a synthetic precursor to intracellular cysteine, is the rate-limiting peptide for synthesis of the endogenous antioxidant GSH, and is also a direct scavenger of reactive oxygen species (ROS). It is used clinically as both an antioxidant and a mucolytic agent 17. Pre-treating MDSCs with NAC before transplantation into acutely infarcted mouse myocardium resulted in increased LV contraction, decreased fibrosis, and increased angiogenesis compared to the transplantation of untreated MDSCs 18.
Myoblasts, one of the most abundant cell sources available for tissue repair, have been restricted in their regenerative applications by various limitations including poor cell survival, low spreading of the injected cells, and immune response 19 , 20. It is tempting to speculate that the poor survival of myoblasts is potentially due to their inherently low levels of antioxidants 21. Consequently, we aimed to determine if NAC antioxidant pre-treatment could increase myoblast survival and cardiac repair, as observed with MDSCs. We demonstrated that increasing antioxidant levels positively correlated with early survival of transplanted myoblasts. NAC pre-treatment increased the number of proliferating cardiac cells and decreased cellular apoptosis in the transplanted hearts. At 6 weeks post-infarction, depleting myoblasts of GSH with DEM prior to implantation resulted in reduced functional and structural repair, similar to those of PBS injected hearts; however, the myoblasts pre-treated with NAC antioxidant did not improve cardiac function at 6 weeks, when compared to the untreated myoblasts. These results suggest that despite pretreating myoblasts with NAC to improve their survivability, the cardiac regenerative ability of the myoblasts remained limited, when compared to earlier progenitor cell populations like the MDSCs.
Materials and Methods
Animal studies: The use of animals and the surgical procedures performed in this study were approved by the Institutional Animal Care and Use Committee of the University of Pittsburgh (protocol #0901823). All animal studies conform to the Guide for the Care and Use of Laboratory Animals as published by the National Institute of Health.
Myoblast isolation: Myoblasts were isolated from the skeletal muscle of three week old C57BL mice (Jackson, Bar Harbor, ME, USA) using the modified preplate technique as previously described 22.
Differentiation assay: Myoblasts were plated at 50 cells/mm2 on a 24-well collagen type-I coated plates, and the differentiation assay was performed as described previously 23.
Glutathione levels: Myoblasts were plated at a density of 30 cells/mm2 in a 96 well plate and treated with PM + 100 µM H2O2, PM + 100 µM H2O2 + 50 µM DEM or PM + 100 µM H2O2 + 10 mM NAC for two and a half hours. To detect glutathione levels treated cells were then reacted with PM + 2.5 mM Probenecid (Sigma) + 50 µM Monochlorobimane (MCB) (Invitrogen) for 20 min.
Cell survival under oxidative stress: Myoblasts were plated in PM at 10 cells/mm2 in a 24-well collagen type-I coated plate. Twenty-four hours later, the media was switched to PM with propidium iodide (PI, 1:500, Sigma, St. Louis, MO, USA), or PM containing 375 µM hydrogen peroxide (H2O2) with PI. Each group (unstressed or oxidative stressed) was then divided into one of three treatment groups: PM, PM + 50 µM DEM, or PM + 10 mM NAC. Plates were placed onto a previously described live cell imaging system (LCI, Kairos Instruments, LLC, Pittsburgh, PA, USA) 24.
Myoblast labeling: Myoblasts were retrovirally transduced with a LacZ reporter gene prior to their transplantation 25.
Cell transplantation to infarcted myocardium: Thirty male, NOD-SCID mice at 14-18 weeks of age were used in this study (Jackson, Bar Harbor, ME, USA). Mice were anesthetized during the surgical procedure with 1-1.5% isoflurane in 100% O2. Mice that underwent permanent left descending coronary artery ligation using a 7-0 prolene suture to create myocardial infarction 26 , 27 , 28 and were randomly allocated between the treatment groups (7 mice per group: PBS, untreated myoblasts, and myoblasts treated with either NAC or DEM). Myoblasts were treated with either 10 uM NAC or 50 µM DEM for twenty-four hours and then rinsed with PBS prior to implantation. Five minutes after the creation of the infarction, mice received a 30 µl injection of one of the following: 30 µl phosphate buffered saline (PBS), 3 x 105 NAC-treated myoblasts in PBS, 3 x 105 DEM-treated myoblasts in PBS, or 3 x 105 untreated control cells in PBS. Cells or PBS were injected into the left ventricular free wall five minutes after permanent ligation of the left coronary artery as previously described 29 , 30. Echocardiography was performed at 2 and 6 weeks after cell transplantation as previously described 31 , 32.
Histology and Immunohistochemistry: Previously described techniques were used to stain section for nLacZ 33. Terminal dUPT nick end-labeling (TUNEL) staining was carried out according to the manufacture’s protocol (ApopTag Plus Peroxidase In Situ Apoptosis Detection Kit; Chemicon, Temecula, CA, USA). Mitotic cells were identified by anti-phospho-S10 Histone H3 (1:300 Milipore, Billerica, Massachusetts, USA) with donkey anti-rabbit 594 Alexa Fluor 594 (1:200; Sigma, St. Louis, MO, USA). Masson Modified IMEB Trichrome (IMEB, San Marcos, CA, USA) was performed according to the manufacturer’s guidelines. The sections were assessed for percentage area of collagen, which were normalized to total tissue area using CellProfiler image analysis software (http://www.cellprofiler.org). CD31 positive cells in the infarct area were determined by staining tissue sections with rat anti-CD31 primary antibody (1:300; Sigma, St. Louis, MO, USA) and donkey anti-rat Alexa Fluor 594 secondary antibody (1:300, Sigma, St. Louis, MO, USA).
Microscopy: Microscopy was performed using either a Nikon Eclipse E800 microscope or a Leica DMIRB inverted microscope, both equipped with a Retiga digital cameras.
Statistical analysis: Means and standard errors were calculated for all measured values, and statistical significance between the groups was determined by a 1-way ANOVA (SPSS Inc., Chicago, IL, USA). In the event of a significant ANOVA, the appropriate multiple comparisons test was used for post-hoc analysis (S-N-K).
Results
Modulation of intracellular antioxidants does not affect myoblast differentiation
In vitro antioxidant levels and myogenic differentiation were analyzed in myoblasts treated with the antioxidant NAC and pro-oxidant DEM. There was a significant difference in GSH expression in the NAC-treated group compared to the DEM-treated group (p<0.05, Figure 1A). There was no difference in myogenic differentiation between the groups (Figure 1B).
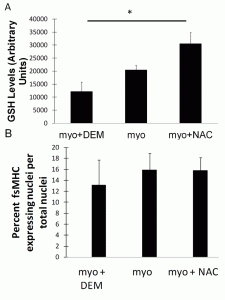
Figure 1. Modulation of antioxidant levels does not affect myogenic differentiation. (A) GSH expression was increased in myoblasts treated with NAC compared to myoblasts treated with DEM (*p<0.05). (B) Differentiation of myoblasts was unchanged after treatment.
NAC pre-treatment increases survival of myoblasts
Myoblasts were treated with NAC or DEM and cultivated under oxidative stress conditions (375 µmol/L H2O2). Treatment of myoblasts with NAC increased cell survival under in vitro oxidative stress conditions at all time points for up to 48 hours following treatment. NAC-treated myoblasts had significantly greater in vitro cell survival than their DEM-treated counterparts (p<0.05, Table 1) but no difference was noted when compared to untreated myoblasts.
To test their in vivo survival, myoblasts were treated with NAC or DEM for 24 hours prior to their transplantation into acutely infarcted hearts. The total number of LacZ+ cells normalized to tissue area was determined 3 days after myoblast implantation (Figure 2A-C). A significantly greater number of LacZ+ myoblasts in the infarct area was observed in the NAC-treated group when compared to the untreated and DEM-treated groups (p<0.05, Figure 2D). Next, cell proliferation was examined in the engraftment area by Histone-3 immunostaining (Figure 2E-G). There were twice as many proliferative cells in the NAC-treated group compared to all other groups (p>0.05, Figure 2H). Cellular apoptosis was examined with TUNEL staining in the engraftment area (Figure 2I-K). Three days after injection, there were significantly less apoptotic cells in the NAC-treated hearts compared to the untreated myoblast controls (p<0.05, Figure 2L). Taken together, these results highlight an initial survival advantage for myoblasts pre-treated with NAC.
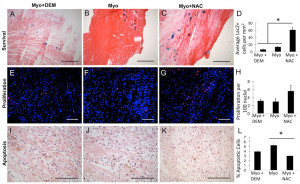
Figure 2. NAC treated myoblasts showed superior survival, increased proliferation, and decreased apoptosis 3 days after transplantation. (A)(B)(C) Representative images of sections stained with LacZ positive myoblasts that were treated with DEM, untreated, or treated with NAC, respectively. (D) There was a significantly greater number of LacZ positive transplanted cells in the hearts injected with myoblasts+NAC compared to myoblasts treated with DEM or untreated myoblasts (*p<0.05) (Scale bar = 0.3 mm). The number of H3 positive cells was normalized to total number of nuclei (DAPI, blue). (E)(F)(G) Representative images of: myo+DEM, myo, and myo+NAC, respectively. (H) The hearts treated with myoblasts+NAC had twice as many proliferating cells as those treated with myo+DEM or untreated myoblasts; however, this was not statistically significant (Scale bar = 0.1 mm). (I)(J)(K) Representative images of sections stained with TUNEL that were transplanted with myoblasts treated with DEM, untreated, or treated with NAC, respectively. (L) There was significantly less apoptosis in the samples treated with myo+NAC as compared to myo (*p<0.05) (Scale bar = 0.3 mm).
Modulation of antioxidants in myoblasts affects functional recovery following MI
Contractile functional parameters were analyzed by echocardiography at 2 and 6 weeks post-treatment. Fractional shortening (FS) at 6 weeks was significantly increased in the NAC-treated group compared to PBS (p<0.05, Figure 3A). Fractional area change (FAC), at 6 weeks, was significantly greater in the NAC-treated group than the DEM-treated and PBS groups while the untreated myoblast group also had significantly greater FAC than the PBS group (p<0.05, Figure 3B). No difference in cardiac function was noted between NAC treated myoblasts and untreated myoblasts at both of the time points tested. As expected, when antioxidants were depleted by DEM, the functional outcomes of myoblast transplantation were no different from the PBS controls.
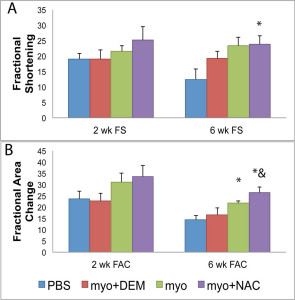
Figure 3. Cardiac Function at 2 and 6 weeks post transplantation. (A) There was no difference in fractional shortening between the groups at 2 weeks; however, at six weeks the myo+NAC treatment group had significantly increased FS compared to PBS (*p<0.05 compared with PBS injection), but no difference was noted when compared to the untreated myoblasts. (B) There was no difference in fractional area change at 2 weeks. At 6 weeks, the myo+NAC treatment group had significantly increased FAC compared to PBS and the myo+DEM and the myo groups were significantly greater than the PBS control (*p<0.05 compared with PBS, & p<0.05 compared with myo+DEM injection, but no difference was noted when compared to the untreated myoblasts.
Depleting Antioxidants in Myoblasts Increases Fibrosis Formation in Treated Hearts
Total fibrotic area was determined by calculating percent fibrosis compared to total tissue area (Figure 4A-D). The myo+NAC and untreated myoblast groups contained significantly less fibrosis in comparison to the myo+DEM and PBS treated groups (*p<0.05, Figure 4E). There was no difference between the groups treated with PBS and myoblasts+DEM and there was no significant difference between the NAC treated and untreated myoblast groups. Depleting antioxidants in the myoblasts before transplantation resulted in increased cardiac fibrosis; however, there was no effect on fibrosis between the NAC treated and untreated myoblast groups.
Depleting Antioxidants in Myoblasts Decreases Angiogenesis in Treated Hearts
In vivo angiogenesis at 6 weeks following cell transplantation was revealed by performing immunohistochemistry to detect the endothelial cell marker CD31 in the peri-infarct area (Figure 4F-I). Quantification of angiogenesis was performed by measuring the number of CD31-positive cells per square millimeter of tissue area. The injected hearts with myo and myo+NAC groups both contained significantly more CD31-positive cells than the PBS and myo+DEM groups (*p<0.05, Figure 4J). There was no significant difference between the NAC-treated and untreated myoblast groups or between the PBS and DEM-treated groups. Depleting antioxidant levels prior to transplantation reduced the donor cells’ ability to induce angiogenesis in the host tissue; however, pre-treating myoblasts with NAC did not further enhance their ability to promote angiogenesis, when compared to untreated myoblasts.
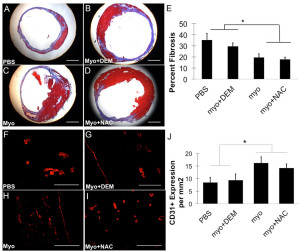
Figure 4. Fibrosis and CD31 positive structures in injected hearts at 6 weeks post implantation. Masson’s Trichrome stains collagen (blue) and muscle (red). Representative images of hearts injected with (A) PBS, (B) myo+DEM, (C) myo, or (D) myo+DEM are shown (Scale bar = 1.0 mm). (E) Percent fibrosis was measured by quantifying the collagen staining compared to the total area. The hearts injected with PBS and myo+DEM had more fibrosis compared to the hearts injected with myo+NAC and myo (*p<0.05). Angiogenesis was measured in the peri-infarct area at 6 weeks by counting the number of CD31 positive cells and normalizing to the tissue area. Representative images of the groups are shown: (F) PBS, (G) myo+DEM, (H), myo, (I) myo+NAC (Scale bar = 0.1 mm). (J) The hearts injected with myo and myo+NAC had significantly more CD31 positive cells than the hearts injected with PBS or myo+DEM (*p<0.05).
Discussion
Myoblasts are an abundant cell source with the potential to induce cardiac regeneration, but they are hindered by numerous limitations including poor survivability, low spreading ability, and the fact that they trigger an intense immune response 34 , 35. We have previously shown that MDSCs repaired infarcted hearts of mice to a greater extent than myoblasts, which we thought to be due to their increased survivability and antioxidant capacity. In fact, we demonstrated that antioxidant pre-treatment of MDSCs further improved the MDSC’s regenerative potential, while depleting the antioxidant reduced their regenerative potential 36 , 37 , 38. We hypothesized that pre-treating myoblasts with NAC would increase the myoblasts’ cell survival and regenerative capacities, making them an equally-effective therapeutic cell source as the rare MDSCs, and that decreasing intracellular antioxidant levels would reduce their regeneration potential.
To determine whether antioxidant pre-treatment would increase the potency of myoblasts for cardiac repair, we treated myoblasts with NAC (to increase antioxidant levels) or DEM (to deplete antioxidant levels) for twenty-four hours before transplanting them into an acute mouse MI model. Three days after transplantation, NAC pre-treatment resulted in increased survival of the transplanted cells compared to untreated myoblasts and DEM-treated myoblasts. We also observed increased cell proliferation and decreased apoptosis in hearts injected with NAC-treated myoblasts compared to the untreated myoblast group.
Oxidative stress has been widely implicated as a mediator of apoptosis. Oxidative stress can be caused by improper ROS elimination and/or an increase in the amount of ROS. Antioxidants help to remove excess ROS from the cell. NAC has dual roles as an antioxidant: as a precursor to intracellular cysteine and GSH and directly as a scavenger of ROS. Antioxidants reduce ROS levels and increase survival in many cell types including: cardiomyoblasts 39, endothelial cells 40, mesenchymal stem cells 41 and muscle-derived stem cells 42 , 43.
Although at 3 days post-transplantation NAC treatment had a positive effect on myoblast survival, by 2 and 6 weeks no significant improvement in cardiac function could be observed when compared to the untreated myoblasts. As expected, the NAC treated cells produced a significantly greater LV contractility in the MI injured hearts when compared to DEM-treated myoblasts or PBS injection group. Levels of myocardial fibrosis and CD31-positive endothelial cells were also similar between NAC-treated and the untreated myoblast group. On the other hand, depleting glutathione in myoblasts with DEM dramatically reduced their regenerative capacity to the level of PBS, similar to DEM treated MDSCs 44 , 45. Our data suggest that maintaining endogenous antioxidant levels is important for the therapeutic outcome of cell therapy utilizing muscle-derived donor cells; however, unlike MDSCs 46 , 47, increasing antioxidant levels in myoblasts was not sufficient at further augmenting the therapeutic effect of the myoblasts on long-term cardiac function. This is also indicative of an intrinsic cellular difference that exists between myoblasts and MDSCs other than their ability to survive because of their antioxidant content. The MDSCs must be producing paracrine factors that have other beneficial effects on improving cardiac function that the myoblasts simply do not secrete or secrete in sufficient amounts.
Another possibility is that the duration of NAC protection in the myoblasts is not persistent, and that a longer sustained exposure to NAC or other survival factors may increase the cardiac regenerative potential of myoblasts; however, the effects of NAC are transient and may simply be insufficient to induce long-term survival benefits in myoblasts. It also suggests that an early increase in survival does not necessarily translate into functional recovery at later time points. Therefore, rigorous testing with multiple time points is required to determine the true effectiveness of cellular therapies.
One way to achieve a more sustained survival of transplanted cells is through gene therapy 48. Previous work has shown that mesenchymal stem cells (MSCs) transduced with the survival factors Akt, the anti-apoptotic Bcl-2 gene, or the hypoxia-regulated heme oxygenase-1 vector improved heart repair after MI compared to control MSCs due to decreased apoptosis and increased cellular survival 49 50 , 51. Future studies with myoblasts could include transducing cells with antioxidant or survival genes to garner a sustained increase in antioxidant capacity; consequently, the beneficial effect of treated myoblasts in cardiac repair might be more pronounced.
The results of this study highlight the fact that early cell survival is not completely indicative of sustained functional cardiac repair. Although there was a larger number of surviving transplanted myoblasts and fewer apoptotic cells in the cardiac tissue of NAC-treated myoblasts at 3 days, at 6 weeks this group did not have significant LV contractile differences than hearts implanted with non-treated myoblasts. Another likely interpretation is that cell survival is not the only factor to be considered for cellular cardiomyoplasty. Although myoblasts treated with NAC have improved survival in the short term, they may not be as capable of preserving cardiac muscle and improving functional performance as more undifferentiated stem cells such as MDSCs. Despite the fact that NAC pre-treatment imparted an early survival advantage on myoblasts, this did not affect the cells ability to proliferate and migrate, or change their growth factor secretion profile. Paracrine factors are known to be important for cardiac repair 52, and myoblasts may secrete different factors than MDSCs, which may partially explain why antioxidant pre-treatment of MDSCs resulted in improved cardiac repair while the same treatment of the myoblasts did not. Moreover, MDSCs are known to have a greater proliferative ability than myoblasts 53 and secrete more VEGF 54. It is likely that more than one aspect of cellular functionality needs to be targeted in myoblasts in order to significantly improve their ability to repair the heart after ischemic injury. Finally, we conclude that early donor cell survival is not indicative of long-term functional benefits in the heart and that the cell source is as important as cell survival to achieve long-term preservation of cardiac function following cellular therapy.
Conflict of Interests
Johnny Huard received consulting fees and royalty payments from Cook MyoSite, Inc. during the period of time in which this project was performed.
Acknowledgments
The authors wish to thank Mitra Lavasani, Jonathan Proto and James Cummins for their excellent editorial assistance with this manuscript. This projected was supported in part by an NIH grant to J.H. (U54AR050733), the Henry J. Mankin Endowed Chair at the University of Pittsburgh, and the William F. and Jean W. Donaldson Endowed Chair at the Children’s Hospital of Pittsburgh and by a predoctoral fellowship to S.B. (T32-HL076124-04).
References
- WRITING GROUP MEMBERS, Lloyd-Jones D, Adams RJ, Brown TM, Carnethon M, Dai S, De Simone G, Ferguson TB, Ford E, Furie K, Gillespie C, Go A, Greenlund K, Haase N, Hailpern S, Ho PM, Howard V, Kissela B, Kittner S, Lackland D, Lisabeth L, Marelli A, McDermott MM, Meigs J, Mozaffarian D, Mussolino M, Nichol G, Roger VL, Rosamond W, Sacco R, Sorlie P, Roger VL, Thom T, Wasserthiel-Smoller S, Wong ND, Wylie-Rosett J; American Heart Association Statistics Committee and Stroke Statistics Subcommittee. Heart disease and stroke statistics—2010 update: a report from the American Heart Association. Circulation 2010; 121(7): e46-e215. (back)
- Payne TR, Oshima H, Okada M, Momoi N, Tobita K, Keller BB, Peng H, Huard J. A relationship between vascular endothelial growth factor, angiogenesis, and cardiac repair after muscle stem cell transplantation into ischemic hearts. J Am Coll Cardiol 2007; 50(17): 1677-1684. (back)
- Li G, Corsi-Payne K, Zheng B, Usas A, Peng H, Huard J. The dose of growth factors influences the synergistic effect of vascular endothelial growth factor on bone morphogenetic protein 4-induced ectopic bone formation. Tissue Eng Part A 2009; 15(8): 2123-2133. (back)
- Robey TE, Saiget MK, Reinecke H, Murry CE Systems approaches to preventing transplanted cell death in cardiac repair. J Mol Cell Cardiol 2008; 45(4): 567-581. (back)
- Laflamme MA, Murry CE. Regenerating the heart. Nat Biotechnol 2005; 23(7): 845-856. (back)
- Zhang M, Methot D, Poppa V, Fujio Y, Walsh K, Murry CE. Cardiomyocyte grafting for cardiac repair: graft cell death and anti-death strategies. J Mol Cell Cardiol 2001; 33(5): 907-921. (back)
- Segers VF, Lee RT. Stem-cell therapy for cardiac disease. Nature 2008; 451(7181): 937-942. (back)
- Saparov A, Chen CW, Beckman SA, Wang Y, Huard J. The role of antioxidation and immunomodulation in postnatal multipotent stem cell-mediated cardiac repair. Int J Mol Sci 2013; 14(8): 16258-16279. (back)
- Gharaibeh B, Lu A, Tebbets J, Zheng B, Feduska J, Crisan M, Peault B, Cummins J, Huard J. Isolation of a slowly adhering cell fraction containing stem cells from murine skeletal muscle by the preplate technique. Nat Protoc 2008; 3(9): 1501-1509. (back)
- Qu-Petersen Z, Deasy B, Jankowski R, Ikezawa M, Cummins J, Pruchnic R, J. Mytinger, Cao B, Gates C, Wernig A, Huard J. Identification of a novel population of muscle stem cells in mice: potential for muscle regeneration. J Cell Biol 2002; 157(5): 851-864. (back)
- Oshima H, Payne TR, Urish KL, Sakai, Ling Y, Gharaibeh B, Tobita K, Keller BB, Cummins JH, Huard J. Differential myocardial infarct repair with muscle stem cells compared to myoblasts Mol Ther 2005; 12(6): 1130-1141. (back)
- Oshima H, Payne TR, Urish KL, Sakai, Ling Y, Gharaibeh B, Tobita K, Keller BB, Cummins JH, Huard J. Differential myocardial infarct repair with muscle stem cells compared to myoblasts Mol Ther 2005; 12(6): 1130-1141. (back)
- Payne TR, Oshima H, Sakai T, Ling Y, Gharaibeh B, Cummins J, Huard J. Regeneration of dystrophin-expressing myocytes in the mdx heart by skeletal muscle stem cells. Gene Ther 2005; 12(16): 1264-1274. (back)
- 13 (back)
- Urish KL, Vella JB, Okada M, Deasy BM, Tobita K, Keller BB, Cao B, Piganelli JD, Huard J. Antioxidant levels represent a major determinant in the regenerative capacity of muscle stem cells Mol Biol Cell 2009; 20(1): 509-520. (back)
- Drowley L, Okada M, Beckman S, Vella J, Keller B, Tobita K, Huard J. Cellular antioxidant levels influence muscle stem cell therapy Mol Ther 2010; 18(10): 1865-1873. (back)
- Dodd S, Dean O, Copolov DL, Malhi GS, Berk M N-acetylcysteine for antioxidant therapy: pharmacology and clinical utility Expert Opin Biol Ther 2008; 8(12): 1955-1962. (back)
- Drowley L, Okada M, Beckman S, Vella J, Keller B, Tobita K, Huard J. Cellular antioxidant levels influence muscle stem cell therapy Mol Ther 2010; 18(10): 1865-1873. (back)
- Oshima H, Payne TR, Urish KL, Sakai, Ling Y, Gharaibeh B, Tobita K, Keller BB, Cummins JH, Huard J. Differential myocardial infarct repair with muscle stem cells compared to myoblasts Mol Ther 2005; 12(6): 1130-1141. (back)
- Menasche P. Skeletal myoblasts and cardiac repair J Mol Cell Cardiol 2008; 45(4): 545-553. (back)
- Menasche P. Skeletal myoblasts and cardiac repair J Mol Cell Cardiol 2008; 45(4): 545-553. (back)
- Gharaibeh B, Lu A, Tebbets J, Zheng B, Feduska J, Crisan M, Peault B, Cummins J, Huard J. Isolation of a slowly adhering cell fraction containing stem cells from murine skeletal muscle by the preplate technique. Nat Protoc 2008; 3(9): 1501-1509. (back)
- Drowley L, Okada M, Beckman S, Vella J, Keller B, Tobita K, Huard J. Cellular antioxidant levels influence muscle stem cell therapy Mol Ther 2010; 18(10): 1865-1873. (back)
- Schmidt BT, Feduska JM, Witt AM, Deasy BM. Robotic cell culture system for stem cell assays. Industrial Robot-an Int J 2008; 35(2): 116-124. (back)
- Payne TR, Oshima H, Sakai T, Ling Y, Gharaibeh B, Cummins J, Huard J. Regeneration of dystrophin-expressing myocytes in the mdx heart by skeletal muscle stem cells. Gene Ther 2005; 12(16): 1264-1274. (back)
- Payne TR, Oshima H, Okada M, Momoi N, Tobita K, Keller BB, Peng H, Huard J. A relationship between vascular endothelial growth factor, angiogenesis, and cardiac repair after muscle stem cell transplantation into ischemic hearts. J Am Coll Cardiol 2007; 50(17): 1677-1684. (back)
- Oshima H, Payne TR, Urish KL, Sakai, Ling Y, Gharaibeh B, Tobita K, Keller BB, Cummins JH, Huard J. Differential myocardial infarct repair with muscle stem cells compared to myoblasts Mol Ther 2005; 12(6): 1130-1141. (back)
- Drowley L, Okada M, Payne TR, Botta GP, Oshima H, Keller BB, Tobita K, Huard J. Sex of muscle stem cells does not influence potency for cardiac cell therapy Cell Transplant 2009; 18(10): 1137-1146. (back)
- Oshima H, Payne TR, Urish KL, Sakai, Ling Y, Gharaibeh B, Tobita K, Keller BB, Cummins JH, Huard J. Differential myocardial infarct repair with muscle stem cells compared to myoblasts Mol Ther 2005; 12(6): 1130-1141. (back)
- Drowley L, Okada M, Beckman S, Vella J, Keller B, Tobita K, Huard J. Cellular antioxidant levels influence muscle stem cell therapy Mol Ther 2010; 18(10): 1865-1873. (back)
- Payne TR, Oshima H, Okada M, Momoi N, Tobita K, Keller BB, Peng H, Huard J. A relationship between vascular endothelial growth factor, angiogenesis, and cardiac repair after muscle stem cell transplantation into ischemic hearts. J Am Coll Cardiol 2007; 50(17): 1677-1684. (back)
- Oshima H, Payne TR, Urish KL, Sakai, Ling Y, Gharaibeh B, Tobita K, Keller BB, Cummins JH, Huard J. Differential myocardial infarct repair with muscle stem cells compared to myoblasts Mol Ther 2005; 12(6): 1130-1141. (back)
- Qu-Petersen Z, Deasy B, Jankowski R, Ikezawa M, Cummins J, Pruchnic R, J. Mytinger, Cao B, Gates C, Wernig A, Huard J. Identification of a novel population of muscle stem cells in mice: potential for muscle regeneration. J Cell Biol 2002; 157(5): 851-864. (back)
- Qu-Petersen Z, Deasy B, Jankowski R, Ikezawa M, Cummins J, Pruchnic R, J. Mytinger, Cao B, Gates C, Wernig A, Huard J. Identification of a novel population of muscle stem cells in mice: potential for muscle regeneration. J Cell Biol 2002; 157(5): 851-864. (back)
- Oshima H, Payne TR, Urish KL, Sakai, Ling Y, Gharaibeh B, Tobita K, Keller BB, Cummins JH, Huard J. Differential myocardial infarct repair with muscle stem cells compared to myoblasts Mol Ther 2005; 12(6): 1130-1141. (back)
- Oshima H, Payne TR, Urish KL, Sakai, Ling Y, Gharaibeh B, Tobita K, Keller BB, Cummins JH, Huard J. Differential myocardial infarct repair with muscle stem cells compared to myoblasts Mol Ther 2005; 12(6): 1130-1141. (back)
- Urish KL, Vella JB, Okada M, Deasy BM, Tobita K, Keller BB, Cao B, Piganelli JD, Huard J. Antioxidant levels represent a major determinant in the regenerative capacity of muscle stem cells Mol Biol Cell 2009; 20(1): 509-520. (back)
- Drowley L, Okada M, Beckman S, Vella J, Keller B, Tobita K, Huard J. Cellular antioxidant levels influence muscle stem cell therapy Mol Ther 2010; 18(10): 1865-1873. (back)
- Rodriguez-Porcel M, Gheysens O, Paulmurugan R, Chen IY, Peterson KM, Willmann JK, Wu JC, Zhu X, Lerman LO, Gambhir SS. Antioxidants improve early survival of cardiomyoblasts after transplantation to the myocardium Mol Imaging Biol 2010; 12(3): 325-334. (back)
- Voghel G, Thorin-Trescases N, Farhat N, Mamarbachi AM, Villeneuve L, Fortier A, Perrault LP, Carrier M, Thorin E. Chronic treatment with N-acetyl-cystein delays cellular senescence in endothelial cells isolated from a subgroup of atherosclerotic patients Mech Ageing Dev 2008; 129(5): 261-270. (back)
- Song H, Cha MJ, Song BW, Kim IK, Chang W, Lim S, Choi EJ, Ham O, Lee SY, Chung N, Jang Y, Hwang KC. Reactive oxygen species inhibit adhesion of mesenchymal stem cells implanted into ischemic myocardium via interference of focal adhesion complex Stem Cells 2010; 28(3): 555-563. (back)
- Urish KL, Vella JB, Okada M, Deasy BM, Tobita K, Keller BB, Cao B, Piganelli JD, Huard J. Antioxidant levels represent a major determinant in the regenerative capacity of muscle stem cells Mol Biol Cell 2009; 20(1): 509-520. (back)
- Drowley L, Okada M, Beckman S, Vella J, Keller B, Tobita K, Huard J. Cellular antioxidant levels influence muscle stem cell therapy Mol Ther 2010; 18(10): 1865-1873. (back)
- Urish KL, Vella JB, Okada M, Deasy BM, Tobita K, Keller BB, Cao B, Piganelli JD, Huard J. Antioxidant levels represent a major determinant in the regenerative capacity of muscle stem cells Mol Biol Cell 2009; 20(1): 509-520. (back)
- Drowley L, Okada M, Beckman S, Vella J, Keller B, Tobita K, Huard J. Cellular antioxidant levels influence muscle stem cell therapy Mol Ther 2010; 18(10): 1865-1873. (back)
- Urish KL, Vella JB, Okada M, Deasy BM, Tobita K, Keller BB, Cao B, Piganelli JD, Huard J. Antioxidant levels represent a major determinant in the regenerative capacity of muscle stem cells Mol Biol Cell 2009; 20(1): 509-520. (back)
- Drowley L, Okada M, Beckman S, Vella J, Keller B, Tobita K, Huard J. Cellular antioxidant levels influence muscle stem cell therapy Mol Ther 2010; 18(10): 1865-1873. (back)
- Mohsin S, Siddiqi S, Collins B, Sussman MA. Empowering adult stem cells for myocardial regeneration Circ Res 2011; 109(12): 1415-1428. (back)
- Mangi AA, Noiseux N, Kong D, He H, Rezvani M, Ingwall JS, Dzau VJ. Mesenchymal stem cells modified with Akt prevent remodeling and restore performance of infarcted hearts Nat Med 2003; 9(9): 1195-1201. (back)
- Li W, Ma N, Ong LL, Nesselmann C, Klopsch C, Ladilov Y, Furlani D, Piechaczek C, Moebius JM, Lützow K, Lendlein A, Stamm C, Li RK, Steinhoff G. Bcl-2 engineered MSCs inhibited apoptosis and improved heart function. Stem Cells 2007; 25(8): 2118-2127. (back)
- Tang YL, Tang Y, Zhang YC, Qian K, Shen L, Phillips MI Improved graft mesenchymal stem cell survival in ischemic heart with a hypoxia-regulated heme oxygenase-1 vector. J Am Coll Cardiol 2005; 46(7): 1339-1350. (back)
- Gnecchi M, Zhang Z, Ni A, Dzau VJ. Paracrine mechanisms in adult stem cell signaling and therapy. Circ Res 2008; 103(11): 1204-1219. (back)
- Qu-Petersen Z, Deasy B, Jankowski R, Ikezawa M, Cummins J, Pruchnic R, J. Mytinger, Cao B, Gates C, Wernig A, Huard J. Identification of a novel population of muscle stem cells in mice: potential for muscle regeneration. J Cell Biol 2002; 157(5): 851-864. (back)
- Oshima H, Payne TR, Urish KL, Sakai, Ling Y, Gharaibeh B, Tobita K, Keller BB, Cummins JH, Huard J. Differential myocardial infarct repair with muscle stem cells compared to myoblasts Mol Ther 2005; 12(6): 1130-1141. (back)
To cite this article
The Cardiac Regenerative Potential of Myoblasts Remains Limited Despite Improving their Survival Via Antioxidant Treatment
CellR4 2014; 2 (2): e845
Publication History
Published online: 31 Mar 2014

This work is licensed under a Creative Commons Attribution-NonCommercial-ShareAlike 4.0 International License.